Posted:
16 Dec 2022
The 2022 Eden Oration was delivered by Professor Edmund Kunji, Fellow in Biology, on 2 December.
At the Eden Feast, we remember the life of Dr Eden, who became Master of Trinity Hall in 1626. On this night, we read the Will of Dr Eden, who became a generous benefactor of this poor college. The Will is a legal document that expresses a person’s wishes as to how their assets are to be distributed after their death, because death is understood to be permanent. Shakespeare said it beautifully: โTo that undiscovered country from whose bourne no traveler returnsโ (Hamlet, Act 3).
What happens with a body after death has been understood for a very long time. โDe terra facta sunt, et in terram pariter revertunturโ, which translates as โAll are of the dust, and all turn to dust againโ (Ecclesiastes 3:20). So long before Chemistry was a subject, it was understood that our bodies are made of the elements of the earth and after death, we fall apart, and the elements are returned to the earth. This process actually follows one of the natural laws of the Universe, which scientists know as Entropy, which is a measure of disorder in the system. Left unchecked, disorder increases over time, and systems dissolve into chaos. Thus, our bodies after death fall apart and disperse following this universal path, but this realisation points to something really important about life.
Life is special, as it seems to cheat this natural process towards greater chaos. Life is organised in compartments, called cells, which are structured and ordered, which can be organised further into organs and bodies. Living organisms are able to synthesise larger molecules, such as DNA, from smaller ones, increasing order. In this way, by structuring and organising, living creatures are able to grow rather than to fall apart. They are able to generate conditions for themselves that are different from that of their environments to control the cellular processes. Living organisms are able to move with purpose, rather than to scatter, like dust in the wind. Thus, life is opposing the path to chaos, but the key question is: what makes that possible? The answer is energy. Energy allows living organisms to structure, to grow and to move. But where does the energy of life come from?
There are some chemical reactions in dark clefts of the earth that can generate small amounts of energy, but the biggest source of energy on earth by far is the sun. Plants and algae can use sunlight as a source of energy to synthesis compounds from smaller components, which is called photosynthesis. For instance, they can use water and carbon dioxide, the gas we breathe out, to synthesise sugar molecules, which in turn can be used to synthesise starch, as food supply, or cellulose, as building material for cell walls and other structural elements. In this way, they synthesise nearly everything they need to form ordered structures that are alive.
Like many other organisms on this earth, we do not have the capacity to use the energy from the sun. It is a fact of life that we need to get energy from the food we eat, which is called metabolic energy. This means we have to eat plants or algae or the animals that eat plants or algae, or the animals that eat other animals. This is why it is such a dog-eat-dog world, as most living creatures need to find a source of food, as a source of metabolic energy.
But how do we extract metabolic energy out of food? This is a very complex biological process, subject of a large part of the Tripos, but I will do it in 10 min, so hold on your benches.
First, we need to take a closer look at ourselves. We always think of ourselves as whole beings, but in reality, we are but a collection of cells. The latest estimates suggest that our body consists of 37 trillion cells. These cells are differentiated, meaning they have different functions and are present in different tissues. For example, there are skin cells, liver cells, brain cells, nerve cells, blood cells and muscle cells, each with a specialised function, but their basic structure is pretty much the same. Surprisingly, each of these cells in our body has to find its own source of metabolic energy to continue living. Thankfully, our differentiated cells have evolved into many organs that help us to find and process food, such as eyes and a nose to detect food, hands to grab food and bring it to our mouth, teeth and molars to cut and grind it, a digestive track to break food down into molecules, which are then taken up into the bloodstream. The heart then pumps these food molecules with our blood all around our body, so that all of our cells, in every remote part of our body, can get the food molecules they need to sustain and energise themselves. So, the cells in our body have both an individual purpose, a specialised function, and common purpose, as they all need to work together to survive collectively. I am confident this is not quite how you have viewed yourself before, but this is how I view you.
How can we eat such a huge variety of different things, as we will enjoy tonight, and get the metabolic energy we need? To get a better understanding, we have to zoom in further and look at the interior of our cells. It turns out that all of our cells, except red blood cells, contain small specialised compartments with specific roles, which we call organelles. There is a nucleus, where our genetic material is stored; an endoplasmic reticulum, the factory of the cell; a Golgi apparatus, the post office of the cell, but the most abundant organelles are called mitochondria. The name derives from the Greek words mitos” (thread) and “chondros” (granule), because of their thread-like appearances. There are about 1500 mitochondria in each cell and they form large and dynamic networks; they can split, they can fuse and they can move around the cell. They have many different roles, but they are best known as the powerhouses of the cell, because of their ability to generate metabolic energy out of food molecules.
To understand how mitochondria work, we need to zoom in further. We have now arrived at the atomic scale, which is the domain of proteins and protein complexes. The best way to describe them is that they are tiny molecular machines capable of carrying out specific tasks in the cell.
Mitochondria can take up nearly all of the food molecules to generate a universal cellular fuel called ATP (adenosine triphosphate), which can be used to power thousands of different cellular activities: the contraction of a muscle cell, the impulse of a nerve cell, the growth of a skin cell. Once used in these energy-requiring processes, ATP falls apart in its constituent components, the spent fuel ADP (adenosine diphosphate) and phosphate. So, we have to remake ATP continuously from its components, but how does that work?
When the food molecules come into mitochondria, the bonds that held them together are broken down by enzymes (also molecular machines) and the electrons that were in those bonds are harvested. These electrons are the same as you will find in electricity that we use to power things, so they carry energy. These electrons then pass through the complexes of the respiratory chain to the oxygen that we breathe, which is then converted to water. This is the primary reason why we have to keep breathing, continuously. The complexes of the respiratory chain use this current of electrons to pump protons, which are positively charged particles, out of the mitochondrion. This process charges the mitochondria up, like a capacitor or battery (~200 mV). This form of energy is then used by another molecular machine, called ATP synthase, which converts the spent fuel ADP into the cellular fuel ATP, using phosphate. ATP synthase works like an electromotor, but is powered by protons, using a central rotating axis to position ADP and phosphate in such a way that ATP spontaneously forms. In this way, we degrade a range of different food molecules, such as sugars and fats, and generate the cellular fuel called ATP.
In fact, we need to generate roughly our own body weight in ATP every day to fuel all of our cellular activities. Since we only have about 50 grams of ATP in our body at any one time, we need to remake it continually from the spent fuel ADP and phosphate, which is why we have to keep eating and breathing. Each ATP molecule is used and remade every minute. This explains why death can occur on a minute time scale. If we stop breathing or if we have no more food molecules available, then the process of ATP generation stops, and we will die. If we stop eating, and thus run out of food molecules, we have to start eating ourselves to stay alive. First, we use our stored sugar reserves, then our fat reserves and finally our protein reserves. So, dieting is really a form of cannibalism, which I find deeply objectionable, as you can see.
So, what do we do in all this? Well, I work with a group of fantastic scientists at the MRC Mitochondrial Biology Unit, which has hosted several Trinity Hall members. Together, we are trying to understand on the molecular level how ADP and ATP and the food molecules are transported in and out of mitochondria. The molecular machine involved in the transport of ADP and ATP is called the mitochondrial ADP/ATP carrier. It is embedded in a membrane that surrounds the mitochondrion, which is impermeable to molecules. Thus, the carrier acts like a portal, facilitating the import ADP and export of ATP. But what does it look like?
Well, the Will of Dr Eden does not stipulate the use of PowerPoint presentations, which is an oversight. So, I brought models of the ADP/ATP carrier in two different states, 10 million times enlarged. In reality, they are not bright fluorescent orange like this, but magnolia.
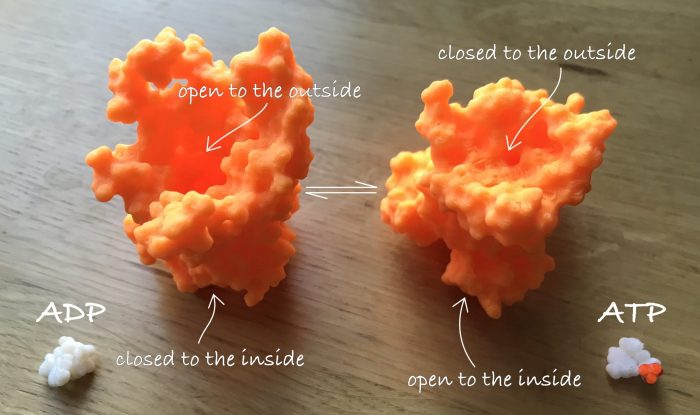
Over a period of twenty years, we worked out how this molecular machine works. The carrier cycles between two states, one that is open to the outside of the mitochondrion and one that is open to the inside of the mitochondrion. The carrier can morph between these two states. So, what happens is that the spent fuel ADP goes into a central cavity and binds there. Then the carrier changes shape and now the carrier is closed to the outside and open to the inside of the mitochondrion. ADP can then diffuse out to ATP synthase, which turns it into ATP. Then, ATP binds to the central cavity, the carrier closes to the inside of the mitochondrion and opens to the outside, and ATP is exported out to fuel our cellular activities. The carrier functions a bit like an airlock in a spaceship, but then on the molecular level.
A rough estimate tells us that we have 50 quintillion (5 with nineteen zeros) of these ADP/ATP carriers in our body and each one can transport 20 ADP and 20 ATP molecules per second. So, when you enjoy your food this evening, think of these hard-working carriers that keep us alive, every moment of our lives for all of our lives.
I would like to close this oration by inviting you to bring your collection of cells over to the Eden Feast, so they can top up their ATP levels, which, after all, keeps you alive! I thank you very much for your attention.
Professor Edmund Kunji is Staff Fellow and Director of Studies in Natural Sciences (Biological), and Research Group Leader, Medical Research Council Mitochondrial Biology Unit. He researches the role of mitochondrial carrier proteins in the transport of metabolites, cofactors and ions across the mitochondrial inner membrane.